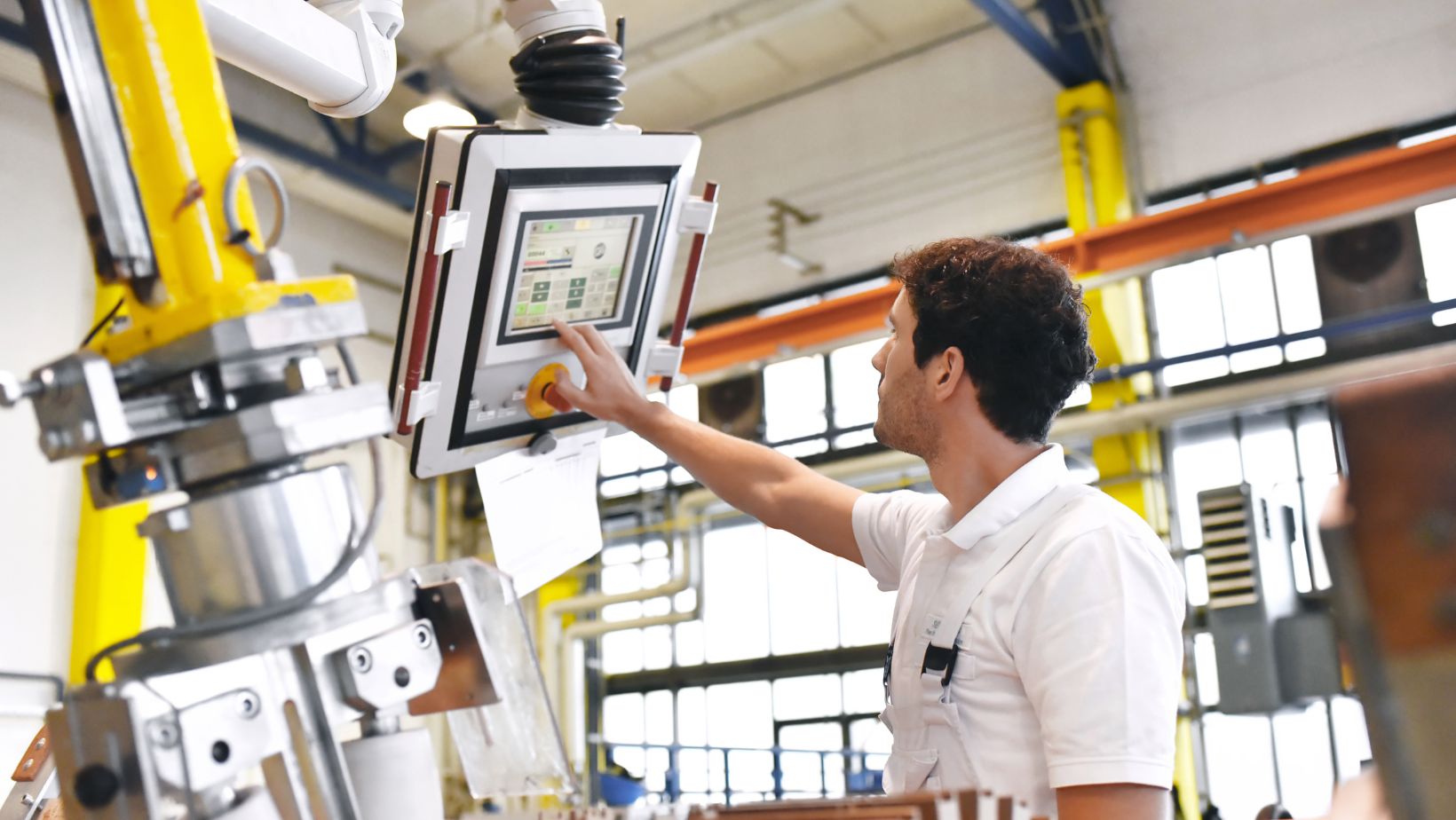
Manufacturing for space, once the domain of speculative science fiction, has transformed into a dynamic field driven by technological advances and increasing commercial interests. The evolution from rudimentary satellite and rocket construction to the sophisticated development of spacecraft and associated technologies represents a significant technological leap. This profound transformation has been marked by innovations in materials, design methodologies, and manufacturing processes tailored for the harsh environment of space.
Early Days of Space Manufacturing
The journey of manufacturing for space began during the mid-20th century with the space race between the United States and the Soviet Union. Initial manufacturing efforts were government-led and focused primarily on constructing robust rockets capable of enduring the stresses of launch and the vacuum of space. Early spacecraft were relatively simple by today’s standards, designed for single uses with limited functionality.
Advances in Materials and Components
As space missions became more ambitious, the limitations of early materials and designs became apparent, prompting significant research and development.
Material Innovations: The extreme conditions of space require materials that can withstand high radiation levels, extreme temperatures, and mechanical stresses. Advances in materials science have led to the development of alloys and composites that are lightweight yet durable enough to protect sensitive electronics and payloads. Materials such as carbon fiber composites and titanium alloys are now staples in spacecraft construction due to their strength-to-weight ratios and resistance to corrosion.
Component Miniaturization and Reliability: The miniaturization of components has been a game-changer in space manufacturing. Modern spacecraft utilize highly reliable, compact systems that integrate functions that once required much larger equipment. This shift not only reduces the weight and cost of spacecraft but also improves their performance and extendibility.
Integration of Advanced Manufacturing Techniques
Additive Manufacturing (3D Printing): One of the most revolutionary advancements in space manufacturing is the adoption of additive manufacturing or 3D printing. This technology allows for the creation of complex components that are often impossible to make with traditional manufacturing methods. 3D printing has dramatically reduced waste, lowered costs, and shortened production cycles for space equipment. It also holds the promise of manufacturing components directly in space, reducing the need for transport from Earth.
Robotics and Automation: The precision required in space manufacturing has made robotics and automation indispensable in the production process. Robots are employed for tasks ranging from the assembly of minute electronic components to the welding of large spacecraft sections, ensuring high precision and consistency.
Drive Controllers and Motors: Essential for automated systems, drive controllers and motors ensure the precise movement and operation necessary in the manufacture of spacecraft. These components are critical for maintaining the stringent tolerances required in space equipment, ensuring that every part operates reliably under extreme conditions
Current Trends and Innovations
Private Sector Involvement: The entry of private companies into space exploration and manufacturing has significantly changed the landscape. Companies like SpaceX, Blue Origin, and others are not only pushing the boundaries of space technology but also driving down costs through innovations in manufacturing processes and reusability.
Sustainability and Reusability: There is an increasing focus on making space travel sustainable. Innovations such as reusable rocket components are a major step forward. Manufacturing processes are now designed to ensure that parts can withstand multiple missions, which involves rigorous testing and the use of highly durable materials.
In-Situ Resource Utilization (ISRU): Looking to the future, ISRU represents a pivotal shift in space manufacturing. The ability to utilize resources from the moon, asteroids, or Mars to build structures or generate fuel could dramatically reduce the costs of space exploration and long-term habitation.
Challenges and Future Directions
Despite tremendous advancements, manufacturing for space faces numerous challenges. The high cost of transporting materials from Earth to space remains a significant hurdle. Additionally, the limited availability of resources in space environments demands continuous innovation in recycling and sustainability practices.
The future of space manufacturing lies in the continued evolution of technologies such as AI and machine learning, which can optimize manufacturing processes and predict maintenance needs. Furthermore, the development of standards for manufacturing interoperability in space will be crucial as international and commercial ventures become more intertwined.
Conclusion
Manufacturing for space has come a long way from its nascent days of bulky rockets and simple satellites. Today, it stands as a testament to human ingenuity, with cutting-edge technologies that push the boundaries of what is possible. As the sector continues to evolve, driven by both technological advancements and strategic international and commercial collaborations, the dream of sustainable and accessible space exploration and habitation draws ever closer to reality. The ongoing refinement and innovation in space manufacturingwill undoubtedly play a critical role in shaping the future of humanity’s endeavors beyond Earth.